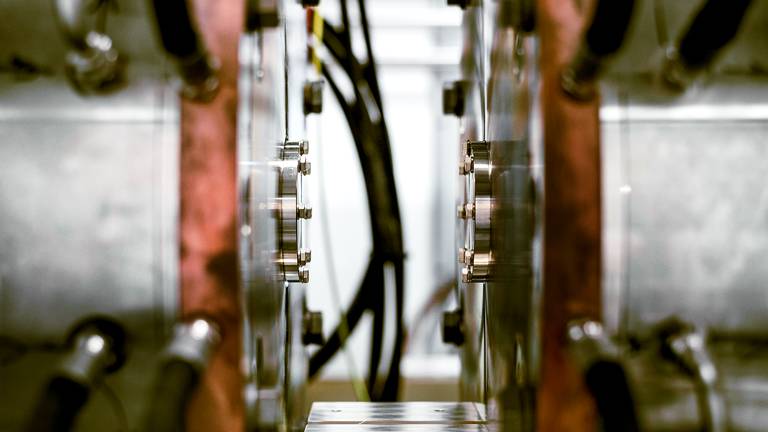
The Large Hadron Collider is the largest and most powerful atom smasher in the world. Built to hunt for new particles and probe the fundamental forces of nature, this massive machine is a 27-kilometer underground loop filled with supercooled magnets and massive detectors that whip particles at the highest speeds possible, to eventually collide into each other.
This field permeates the universe, leading some to suspect that the Higgs may play an important role in the origin of everything. But at this point, the Large Hadron Collider (LHC) and the community that built it are at a crossroads.
Physicists haven't found the supersymmetry particles they were hoping to see. If they did, it would have solved some open mysteries we have about the Higgs and the way the universe works internally.
Scientists anticipated (for 40 years before the Higgs was discovered), that the Higgs could not be a lonely elementary particle. It would have to come along with a lot of other things in order to give a coherent, rational explanation for the origin of its mass.
The big surprise since July 4, 2012, when the Higgs was triumphantly discovered, is no more accompanying particles were discovered. So that's really 4 decades of a certain paradigm for what's going on in physics associated with the Higgs that has not worked out the way theorists had imagined it would.
That's kind of fascinating! I think the last time something of this degree of surprise happened in theoretical physics was a little over 100 years ago.
When you run into a kind of elementary particle you've never seen before, (and you’ve never seen anything like it in physics before) you just put it under a microscope and you study it for the rest of your life. It’s pretty remarkable that we need to build enormous machines that produce an incredible amount of energy to probe the smallest things in the universe.
The push towards higher collision energies to discover new particles is connected to Albert Einstein’s famous equation, E=mc^2. There’s an equivalence here between energy on one side and mass on the other side.
The higher the energy we bring into this collision, the higher the mass of a particle that is forming out of this energy can be. To get more juice out of the machine, CERN shut the LHC down for performance upgrades.
They’re working on cranking up the luminosity. Luminosity is a measure for the quality of a collider. In some sense, it tells you how many collisions per second this collider can provide. When two of the elementary particles have a head-on collision, you can tell that happened because the result of those collisions come out at larger angles relative to the beams.
But it's still an incredibly messy, kind of complicated environment and even when we produce new elementary particles like the Higgs, they don't come out wearing a name tag saying I am a Higgs, they decay in a blink of an eye. It's the results of those decays that experimental colleagues have to sift through like they're looking for a needle in a haystack in order to actually see the evidence.
It will be very beneficial to operate this infrastructure until about 2035 or 2040. By then, we will have collected such a huge amount of data from the collisions that we somehow saturate the knowledge that can be provided by this machine.
Operating it five years longer or 10 years longer will not give significantly more information, which means for particle physicists that the useful time of life of this accelerator will be reached. These time scales seem way out in the future, but to put this in perspective: planning for the Large Hadron Collider began back in the 1980s, construction was approved in 1994 and the first runs didn’t start until 2008. So to prepare for what comes next, teams are delivering conceptual designs for next-generation particle machines.
First thing's first though, CERN wants a bigger tunnel. On a map, you can imagine you have a circle, which is the LHC, and then you would put a new circle that is roughly four times larger. The whole existing CERN accelerator complex, including the LHC, would serve as a pre-accelerator for this future 100 km machine!
Like the gearbox in a car, if you want to drive very fast you must have several gears. You start in a small gear at low velocity, and once you accelerate, you go to the second gear, third gear, fourth gear, fifth gear. This thing is very similar. We would start with small accelerators at low energy, and then we go larger, larger, larger, and to higher energy, higher energy, higher energy.
The CERN study presents a path forward to achieve these energy gear shifts. There’s a new lepton collider, which collides electrons and positrons, a more advanced hadron collider, which collides protons and protons and then heavy ions and then a third option, an electron-proton collider.
When we say the electron looks point-like and the proton does not, it actually means if you bounce things off the electron you see that the way photons bounce off of it, you'll see that the electron has no substructure of any sort. Who knows, if we're probing things with microscopes that are a million times stronger than anything we've seen in some alien civilization that's a million times stronger than the LHC, maybe we would see some substructure to the electron too.
Unlike the electron, a proton is not an elementary particle. The proton is kind of a big messy object that's made up out of smaller constituents known as quarks that are held together inside the proton by the imaginatively named gluons.
When we smash protons into each other at incredibly high energies, one set is going this way at 0.9999999 the speed of light, the others are going the other way the same number of 9s times the speed of light, and when they smash into each other, mostly they go splat. The debris of the collisions goes into the direction of the beams that were coming in.
The next generation Hadron Collider would smash protons together like the LHC, except it'd reach energies of 100 trillion electron volts. The Hadron Collider would provide much higher collision energies that would allow direct creation of, today not known particles. This boosted machine could be used as a tool to search for theoretical particles like WIMPS, which are connected to dark matter.
A factor of 100 in precision is what we need to decisively settle the question of whether the Higgs looks more point-like than anything we've seen before as far as its probes interact with other particles, a factor of 10 higher in energy will let us produce billions of Higgs. 100 TV is what we need to settle this question of the simplest model of weakly interacting particles.
The natural sequence is clearly to start with a lepton collider, which is also a machine that is today technically ready for construction.
In parallel to the operation and physics analysis of this machine, you can use the time to develop the very high-field superconducting magnets that you need for the successor machine.
The magnets currently operating in the LHC tunnel can only reach eight or nine Tesla, which is the magnetic field strength. So we want to double this to 16 or even higher. Magnets are, in this case, the real challenge for such a project. All these things need to be addressed from the very beginning in small setups because you do not want to build 15 meter long heavy magnets every time to test something new.
While this project is an incredible scientific endeavor, the price tag is very steep. These future colliders could cost over $25 billion dollars and would need investment from the international community to even get off the ground. For this decision process, there are several aspects, of course.
There's a scientific-political one, there is an economic one and there is of course also a physics community process.This is exactly what started out as a bottom-up opinion-making process, which is taking place in Europe in the coming year. While the discussions continue, some have even questioned whether an investment like this is even the right course forward for the particle physics community.
There are questions over whether the science case is as strong, if investing in this project is worth the cost compared to other global issues, and how we can be so sure a machine of this magnitude can answer these big questions. There's a spectrum of possibilities for what could be out there theoretically and so we can’t know until we look.
What's definitely true, is that no one who is arguing for building these next machines is now saying we should build them because we expect to see particle x, we should build them because supersymmetry or extra dimensions are around the corner or anything like that since it cannot be guaranteed at all.