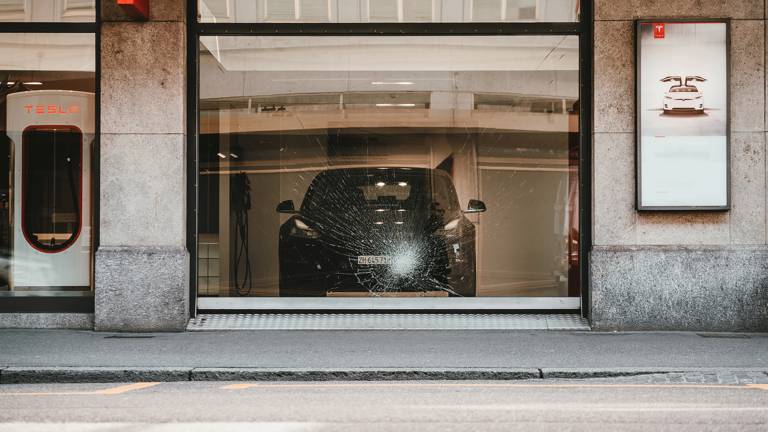
Tesla has grown rapidly over the past decade, becoming the first American auto company to go public since Ford in 1956. The attraction to Tesla is undeniable. Their cars are very smooth, their acceleration is crazy, and perhaps most importantly, the brand represents a direction towards renewable energy.
Tesla has attracted thousands of intelligent people who want to play their part in the world, but for many electric car owners and electric car opponents, there have been some questions.
As Tesla begins to age, Tesla's exotic and demanding models, such as Tesla Truck and Roadster 2 posses their share in the automotive market making these questions more prominent.
The batteries are not cheap, but the price is getting gradually cheaper. With increasing volume of manufacturing and technology advances, the cost per kWh of the Tesla Power Unit and the market average both have a sharp decline but the more the battery is used, the battery storage capacity slowly decreases, reducing the effective range of electric vehicles.
Tesla currently provides warranty to all Mode 3 owners with a warranty range of about 8 years or 160,000 km - whichever comes first.
Under normal use conditions, this warranty ensures that the battery capacity is kept above 70%. If it comes below this value, they will replace your battery for free. It is very difficult to find out what is considered normal, but they seem reasonable and it reports through customer satisfaction.
Tesla estimates that the cost per kW of the battery pack is around $150, so the basic model of the 50 kWh battery pack costs about $7,500 to replace. Considering that price, they must be totally confident about their claimed numbers because recalling the approximately 19 thousand Model 3 will destroy Tesla.
The batteries under guarantee are very unlikely to drop below 70% capacity in less than 160,000 km, but even so, It makes very much sense to work on improving batteries to retain these customers in the future.
This is just one of the reasons behind Tesla's recent acquisition of Maxwell Technologies for $218 million. A Tesla acquisition that brings Tesla not only cheaper batteries, but more optimized batteries. Batteries which will be lighter, have greater range and will last more.
Previous attempts
This is not the first time a car company underestimated its battery degradation. When Nissan Leaf debuted in 2010, the battery production they needed did not exist at all, and the technologists needed to design such battery packs were not available yet. In those days, for notebook-grade batteries, the cost of a lithium-ion battery was $400 per kWh, while for a lithium-ion battery with the life of an electric vehicle, it was as much as $1,000 per kWh.
In order to minimize costs, Nissan decided to produce its own battery and choose a small battery of 24 kWh, making it possible to go just around 100 kilometers which were suitable for city driving.
But customers quickly realized that this range is rapidly decreasing. In just 1-2 years of driving, the Leaf battery capacity dropped up to 27.5% during normal use. Despite careful internal testing, Nissan ignored some of the key test conditions when developing batteries and because they made some key design errors.
What was the problem
To understand why this degradation occurs, you first need to understand how lithium-ion batteries work. Lithium-ion batteries, like all batteries, contain a positive electrode (anode) and a negative electrode (cathode), separated by an electrolyte.
The battery runs the device by transferring positively charged ions between the anode and the cathode, creating an electrical potential between the two sides of the cell and forcing electrons through the device to equalize the potential. Importantly, this process is reversible for lithium-ion batteries because lithium ions are loosely held in the space of the anode and cathode crystal structures.
This is called intercalation. Therefore, when the opposite potential is applied to the battery, it will force the lithium ions back through the electrolytic bridge and place themselves in the anode again.
This process determines a large amount of energy storage capacity of the battery. Lithium is an ideal material for batteries with an atomic number of 3, which is the lightest element of 3r and the lightest metal. Allows its ions to provide incredible energy for the weight characteristics of any battery. However, the energy capacity of the battery is not determined thereby but rather determines how much lithium ions can fit into these spaces in the anode and cathode.
For example, a graphite anode requires carbon atoms to store a single lithium ion to form the molecule (LiC6). This gives the theoretical maximum battery capacity of 372 mAh/gram.
However, you can do better. A single helium atom can bind 4.4 lithium ions to a theoretical maximum battery capacity of 4200 mAh/gram.
This looks great and can provide more battery capacity, but it also has drawbacks. When those 4,4 lithium ions deposited in the silicon crystal lattice they cause 4 times volume expansion for a single full-charge.
This expansion creates stress in the battery, damaging the anode material, and eventually destroys its battery capacity in repeated cycles. Battery designers are always looking for ways to maximize battery energy density without sacrificing battery life.
So what exactly is being damaged in the battery making them lose capacity?
When the researchers started to investigate the cause of the Nissan Leaf's rapid battery degrade, they tried to open the battery and expand the battery content. They found that the electrode coating became rough during its lifetime, and apparently an irreversible reaction occurred within the cell - a change which was expected.
In fact, the chemical process that causes it is critical for the operation of the battery. When the battery is charged for the first time, a chemical reaction occurs at the interface of the electrolysis electrode, in which electrons and ions are combined.
This results in the formation of a new layer between the electrode called the solid electrolyte intermediate (SEI) phase. This name is exactly what it implies, it is a layer formed by the reaction of a liquid electrolyte with an electron to form a solid layer.
Fortunately, this layer is permeable to ions but not to electrons. Therefore, it initially forms a protective layer on the electrode that allows ions to enter while preventing further reaction with the electrolyte. At least this is the idea under normal conditions.
OK, then.. Where is the issue?
The problem is that under certain conditions, the layer can grow beyond the thin layer of the protective coating and cause permanent partitions of lithium. The charging rate and temperature can also accelerate the thickening of the layer.
The temperature at which the battery is charged and discharged plays an important role in battery performance. Lowering the temperature reduces chemical activity, but it is a double-edged sword. Reducing chemical activity affects the ability of the battery to store energy negatively. This is why the battery has a lower range in cold countries. Because of this, in many countries, the Nissan Leaf battery lost its huge capacity in just a few years.
Nissan conducted most of the tests under stable laboratory conditions, not within the possible temperature range. Because of this, they were not aware of the catastrophic effects of temperature on battery life and failed to include thermal management systems, which are commonly used in every Tesla vehicle.
This, of course, will reduce the energy density of the battery. The addition of piping, the glycol required to exchange heat, and the heat pumps and valves required to make the thermal management system not only add weight but also draw energy from the battery to operate. But it plays a vital role in maintaining battery performance. Nissan's set does not include a thermal management system, and even in the 2019 version, it is a bad choice for anyone living in a cold climate.
This is where Maxwell technology comes into play. Maxwell has two main technologies that Tesla will use.
The first is something that Maxwell is famous for: The Ultracapacitor.
Ultracapacitors are used as a basis for battery storage to store energy, but their functions are used in completely different ways for completely different purposes.
The fundamental difference between a capacitor and a battery is that the battery stores energy through a chemical reaction. A lithium-ion battery is produced by introducing a crystal lattice.
Instead, the capacitors store their energy by attaching ions to the surface of the electrode. On each side, we have an aluminum current collector, each with a thin graphite electrode separated by an electrolyte and a separator to prevent electrons from passing through. In the uncharged state, ions float in the electrolyte.
When a voltage is applied during charging, the ions drift toward their opposite charges, thereby adhering to the surface, maintaining the electric charge. Then, when the device is connected to a capacitor, the charge can leave quickly while the ions drift back into the electrolyte.
Nanotechnology has made amazing advances in this area. But even with such a large surface area, Ultracapacitors simply cannot compete with batteries in terms of energy density.
Even though Maxwell's best Ultracapacitors have an energy density of only 7. 4 Wh/kg, Tesla's current best estimate of energy density is about 250 Wh/kg.
Contrary to what the company's technical channels may tell you, Ultracapacitors are not a substitute for batteries. They are designed to work with batteries. The strength of Ultracapacitors is that they can be quickly charged and discharged without damage.
This makes them an excellent buffer for arranging motors and batteries. Their high discharge rate allows current to be supplied to the motor when fast acceleration is required, and they can be quickly charged when disconnected.
This behavior can save battery from unnecessary cycles and increase its ability to provide current quickly when acceleration is required. This will be an essential and powerful technology for the upcoming two Tesla cars.
The second car is a Tesla Truck. Tesla trucks will be limited by their range and cargo transport capacity since it requires heavy-duty batteries because the weight of trucks in most countries is limited to about 40 metric tons. This Ultracapacitor technology will increase its ability to regain energy from breaks, thereby reducing the need to the battery capacity, allowing trucks to swap batteries for cargo.
Maxwell’s second technology has been their next major breakthrough: The dry battery electrode (DBE). This is the manufacturing progress that Maxwell claims will reduce production costs. The factor that with the growth of Gigafactory Tesla has been working hard to minimize.
What is a dry coated battery?
Currently, using Panasonic's patented technology, Tesla dissolves the electrode material in a solvent in order to coat their current collectors with the electrode material, then dispersed on a current collector, and then both are passed through an oven. Drying, in which only the electrode material is left after evaporation of the solvent.
Instead, Maxwell uses adhesives and conductive agents, which are similar to electrostatic coatings. If the painted metal produces a negative charge, the coating will produce a positive charge when it is sprayed and will attract it to the metal, causing it to adhere to the metal.
This painting process also eliminates the solvents required in the coating.
Only time will tell if this can be achieved on an industrial scale. But for Tesla, possessing this production technology is more than scientific research. The cost of having to buy a battery from Panasonic adds to the total cost, and Tesla wants to avoid it in the future. That is a step that allows for complete vertical integration of battery manufacturing. Thereby making the electronic vehicle more suitable for everyday consumers.